Overview of Flex PCB Assembly
Flexible printed circuit board (PCB) assembly, also known as flex circuit assembly or flex PCB assembly, is a specialized manufacturing process that involves mounting electronic components onto a flexible PCB substrate. Flex PCBs are made from thin, flexible materials like polyimide that allow the board to bend and conform to different shapes. This makes them ideal for applications requiring a compact, lightweight, or flexible design.
The flex PCB assembly process shares many similarities with traditional rigid PCB assembly, but there are some key differences and challenges to consider. The flexible nature of the substrate requires specialized handling, soldering techniques, and design considerations to ensure a reliable end product.
In this comprehensive article, we will dive deep into the world of flex PCB assembly. We’ll cover the basics of flex circuits, the advantages they offer, and the various applications where they are used. We’ll also explore the flex PCB assembly process in detail, including the materials, design considerations, manufacturing steps, and quality control measures involved. By the end, you’ll have a thorough understanding of what flex circuit assembly entails and how it enables the creation of innovative electronic products.
What are Flex Circuits?
Flex circuits, also known as flexible printed circuits (FPCs), are a type of PCB made from flexible materials. Unlike traditional rigid PCBs that use a solid fiberglass substrate, flex circuits are built on thin, bendable substrates like polyimide or polyester. This allows them to conform to various shapes and fit into tight spaces where rigid boards cannot.
Flex circuits typically consist of one or more layers of conductive copper traces bonded between layers of flexible insulating material. The number of layers can vary depending on the complexity of the circuit, with single-sided, double-sided, and multi-layer options available. The copper traces are usually etched or printed onto the flexible substrate using methods similar to those used for rigid PCBs.
One of the key characteristics of flex circuits is their ability to bend and flex repeatedly without breaking or losing functionality. This is achieved through the use of specialized materials and design techniques that allow the copper traces to stretch and compress without cracking or delaminating from the substrate. The flexibility of the circuit can be further enhanced by incorporating features like accordion folds, which allow the circuit to expand and contract like a bellows.
Flex circuits offer several advantages over traditional rigid PCBs, including:
- Reduced weight and space requirements
- Increased design flexibility and conformability
- Improved electrical performance due to shorter signal paths
- Enhanced mechanical durability and shock resistance
- Simplified assembly and installation processes
These benefits make flex circuits an attractive choice for a wide range of applications, particularly in industries where size, weight, and flexibility are critical factors.
Applications of Flex Circuit Assemblies
Flex circuit assemblies find use in a diverse array of industries and applications where traditional rigid PCBs may not be suitable. Some common areas where flex PCB assemblies are employed include:
Consumer Electronics
Flex circuits are widely used in consumer electronic devices like smartphones, tablets, laptops, cameras, and wearables. Their thin, lightweight, and flexible nature allows designers to create compact, ergonomic products with increased functionality. Flex circuits enable features like foldable displays, curved screens, and slim form factors that have become increasingly popular in modern devices.
Medical Devices
The medical industry relies heavily on flex circuit assemblies for various applications. Flex circuits are used in implantable devices like pacemakers and defibrillators, where their compact size and biocompatibility are essential. They are also found in medical imaging equipment, surgical instruments, and wearable health monitors. The flexibility of flex circuits allows them to conform to the contours of the human body, making them comfortable to wear and less obtrusive for patients.
Automotive Electronics
Flex PCB assemblies play a crucial role in the automotive industry, where they are used in various electronic systems like engine control modules, infotainment systems, and advanced driver assistance systems (ADAS). The harsh operating environment inside vehicles, with exposure to vibrations, temperature extremes, and moisture, demands robust and reliable electronics. Flex circuits offer improved durability and shock resistance compared to rigid PCBs, making them well-suited for automotive applications.
Aerospace and Defense
The aerospace and defense industries employ flex circuit assemblies in a range of applications, from avionics systems and satellite communications to military equipment and weaponry. Flex circuits offer significant weight savings compared to rigid PCBs, which is crucial in aerospace applications where every gram counts. Their ability to withstand extreme temperatures, vibrations, and G-forces also makes them ideal for the demanding environments encountered in these industries.
Industrial Equipment
Flex PCB assemblies are used in various types of industrial equipment, such as robotics, automation systems, and machine vision devices. The flexibility of flex circuits allows them to be routed through tight spaces and around moving parts, enabling more compact and efficient designs. They also offer improved reliability and durability in the face of the harsh conditions often found in industrial settings.
Application | Benefits of Flex Circuits |
---|---|
Consumer Electronics | Compact size, design flexibility, enhanced functionality |
Medical Devices | Biocompatibility, conformability to body contours, patient comfort |
Automotive Electronics | Durability, shock resistance, reliable performance in harsh environments |
Aerospace and Defense | Weight savings, ability to withstand extreme conditions |
Industrial Equipment | Compact design, routing flexibility, improved reliability |
These are just a few examples of the many applications where flex circuit assemblies are used. As technology continues to advance and the demand for smaller, lighter, and more flexible electronics grows, the use of flex circuits is likely to increase across various industries.
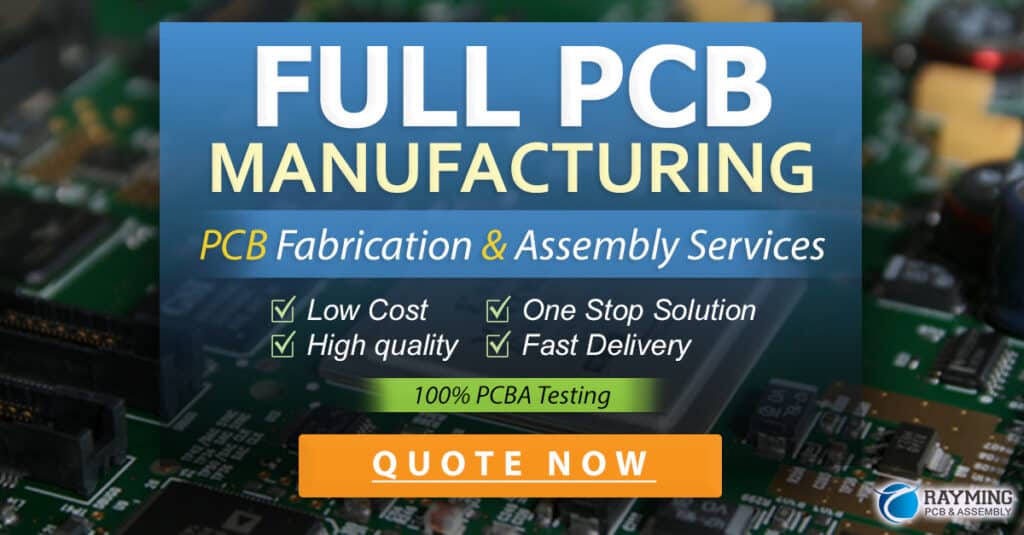
Flex PCB Assembly Process
The flex PCB assembly process involves several steps to transform a bare flex circuit board into a functional electronic assembly. While the overall process is similar to that of rigid PCB assembly, there are some unique considerations and challenges that arise due to the flexible nature of the substrate. Let’s take a closer look at each stage of the flex PCB assembly process.
Flex Circuit Design
The first step in the flex PCB assembly process is designing the circuit. This involves creating a schematic diagram that defines the electrical connections between components, as well as a layout that specifies the physical placement of those components on the board. Flex circuit design requires careful consideration of factors like bend radius, material selection, and layer stackup to ensure the circuit can flex and function as intended.
Designers must also account for the unique mechanical stresses that flex circuits experience during use. This may involve incorporating features like strain relief, z-axis bend areas, and tear stops to prevent damage and improve reliability. Simulation tools are often used to analyze the circuit’s behavior under various bending and flexing scenarios to optimize the design.
Material Selection
Selecting the appropriate materials is crucial for the performance and reliability of a flex circuit assembly. The most common substrate material for flex circuits is polyimide, which offers excellent thermal stability, chemical resistance, and mechanical properties. Other substrate options include polyester, which is lower cost but less durable, and liquid crystal polymer (LCP), which provides superior high-frequency performance.
The conductive traces on a flex circuit are typically made from copper, with various thicknesses and plating options available depending on the application requirements. Adhesives are used to bond the layers of the flex circuit together, with acrylic and epoxy being common choices. Cover layers, stiffeners, and other reinforcement materials may also be incorporated to improve the circuit’s mechanical properties and protect the components.
Fabrication
Once the design is finalized and the materials are selected, the flex circuit is fabricated using a series of photolithography, etching, and lamination processes. The basic steps involved in flex circuit fabrication include:
- Applying a photoresist layer to the copper-clad substrate
- Exposing the photoresist to UV light through a photomask to define the circuit pattern
- Developing the photoresist to remove the unexposed areas
- Etching away the unwanted copper to create the circuit traces
- Stripping away the remaining photoresist
- Laminating the layers of the flex circuit together with adhesives
- Drilling or laser-cutting any necessary holes or apertures
- Applying solder mask and silkscreen layers for protection and labeling
The fabrication process for flex circuits requires specialized equipment and expertise to ensure the delicate materials are handled properly and the circuit meets the required specifications.
Component Assembly
With the flex circuit fabricated, the next step is to assemble the electronic components onto the board. This involves soldering the components to the copper pads using techniques like surface mount technology (SMT), through-hole technology (THT), or a combination of both. Due to the flexible nature of the substrate, special care must be taken to avoid damaging the components or the circuit during handling and soldering.
Automated pick-and-place machines are often used to place SMT components onto the flex circuit with high precision and speed. The circuit is then run through a reflow oven, which melts the solder paste and creates a permanent electrical and mechanical connection between the components and the board. For THT components, manual or wave soldering techniques may be used instead.
Flex circuits may also incorporate additional features like stiffeners, which are attached to certain areas of the circuit to provide local rigidity and support for components. Connectors, cables, and other interface elements are also typically assembled onto the flex circuit at this stage.
Quality Control and Testing
Once the components are assembled, the flex circuit undergoes a series of quality control checks and tests to verify its functionality and reliability. This may include:
- Visual inspection to check for defects like solder bridges, cold joints, or misaligned components
- Automated optical inspection (AOI) to detect any assembly errors or deviations from the design
- X-ray inspection to examine solder joints and other hidden features
- Electrical testing to verify the circuit’s continuity, resistance, and insulation
- Functional testing to ensure the circuit performs as intended in its end-use application
- Environmental testing to validate the circuit’s performance under various operating conditions (e.g., temperature, humidity, vibration)
Any defects or issues identified during quality control are addressed through rework or repair processes. Once the flex circuit assembly passes all the necessary tests, it is ready for integration into the final product or system.
Design Considerations for Flex PCB Assembly
Designing a flex circuit assembly requires careful consideration of various factors to ensure optimal performance, reliability, and manufacturability. Here are some key design considerations to keep in mind when working on a flex PCB assembly project:
Bend Radius
One of the most critical design aspects of a flex circuit is the bend radius, which refers to the minimum radius that the circuit can be bent without causing damage or degrading performance. The bend radius is determined by factors like the substrate material, copper thickness, number of layers, and component placement. Designers must ensure that the bend radius is within the acceptable range for the chosen materials and design parameters.
Tight bend radii can put excessive stress on the copper traces and cause them to crack or delaminate from the substrate. To mitigate this risk, designers can incorporate features like z-axis bend areas, which allow the circuit to flex in a specific direction while minimizing stress on the traces. Tear stops, which are small cut-outs or holes in the substrate, can also be used to prevent cracks from propagating across the circuit.
Material Selection
As mentioned earlier, selecting the appropriate materials is crucial for the performance and reliability of a flex circuit assembly. In addition to the substrate and conductive materials, designers must also consider the properties of adhesives, cover layers, and stiffeners used in the assembly.
For example, the adhesive used to bond the layers of the flex circuit together must be compatible with the substrate material and provide adequate peel strength to prevent delamination. Cover layers, which protect the circuit from environmental factors like moisture and abrasion, must be chosen based on the application requirements and the level of protection needed.
Stiffeners, which are used to provide local rigidity and support for components, must be made from materials that are compatible with the flex circuit and the assembly process. They should also be designed to minimize stress concentrations and ensure proper alignment with the components.
Panelization
Panelization refers to the process of arranging multiple flex circuits on a single panel for fabrication and assembly. This is done to improve efficiency and reduce costs by allowing multiple circuits to be processed simultaneously. However, panelization also introduces some design challenges that must be considered.
Designers must ensure that there is adequate spacing between the individual circuits on the panel to allow for the necessary tooling and handling during fabrication and assembly. They must also consider the panel layout and orientation to minimize waste material and ensure that the circuits can be easily depanelized after assembly.
Panelization can also impact the mechanical stress on the flex circuits during handling and processing. Designers may need to incorporate features like breakaway tabs or perforations to allow the circuits to be easily separated from the panel without causing damage.
Termination and Interconnects
Flex circuits often need to interface with other components, boards, or systems, which requires careful design of termination and interconnect features. This may include connectors, solder pads, or other types of contacts that allow the flex circuit to be electrically and mechanically connected to other elements.
Designers must ensure that the termination and interconnect features are compatible with the mating components and can withstand the mechanical stresses associated with the application. They must also consider factors like contact resistance, signal integrity, and electromagnetic compatibility when designing these features.
In some cases, flex circuits may be designed with built-in connectors or cable assemblies to simplify the integration process. This requires close collaboration between the flex circuit designer and the connector or cable supplier to ensure proper fit and functionality.
Shielding and Grounding
Flex circuits used in high-frequency or noise-sensitive applications may require shielding and grounding features to minimize electromagnetic interference (EMI) and ensure signal integrity. This can be achieved through techniques like incorporating ground planes, using shielded cables or connectors, or applying conductive coatings to the flex circuit.
Designers must consider the placement and routing of ground traces to provide a low-impedance path for return currents and minimize loop areas that can act as antennas. They may also need to incorporate features like vias or conductive gaskets to provide electrical continuity between different layers or sections of the flex circuit.
Shielding and grounding requirements can impact the overall design and layout of the flex circuit, as well as the choice of materials and components used in the assembly. Designers must balance these requirements with other factors like flexibility, cost, and manufacturability to arrive at an optimal solution.
Design Consideration | Key Factors |
---|---|
Bend Radius | Substrate material, copper thickness, number of layers, component placement |
Material Selection | Compatibility, peel strength, protection level, stiffness |
Panelization | Spacing, layout, orientation, depanelization features |
Termination and Interconnects | Compatibility, mechanical stress, contact resistance, signal integrity |
Shielding and Grounding | Ground planes, shielded cables/connectors, conductive coatings, via placement |
By carefully considering these and other design factors, engineers can create flex circuit assemblies that meet the unique requirements of their applications while ensuring optimal performance, reliability, and manufacturability.
Challenges and Solutions in Flex PCB Assembly
While flex PCB assembly offers many benefits, it also presents some unique challenges compared to traditional rigid PCB assembly. Here are some of the key challenges encountered in flex PCB assembly and the solutions used to address them:
Handling and Fixturing
One of the primary challenges in flex PCB assembly is handling the flexible circuits during processing without causing damage or distortion. Unlike rigid PCBs that can be easily fixed in place, flex circuits tend to move and deform when subjected to forces during assembly.
To address this challenge, specialized fixturing and handling equipment is used to keep the flex circuits stable and aligned during processing. This may include custom-designed pallets, trays, or carriers that hold the circuits in place while allowing access for component placement and soldering. Vacuum or mechanical clamping systems may also be used to temporarily fix the circuits in a flat or curved position as needed.
Automated handling systems, such as robotic arms or conveyors, can also be employed to minimize manual handling and reduce the risk of damage. These systems must be carefully designed and programmed to accommodate the unique properties of flex circuits and ensure gentle, precise handling.
Soldering
Soldering components onto a flex circuit can be more challenging than on a rigid PCB due to the potential for thermal damage and mechanical stress. The thin, flexible substrate is more susceptible to warping or distortion when exposed to high temperatures during soldering.
To mitigate these risks, several techniques can be employed. One approach is to use low-temperature soldering processes, such as vapor phase or laser soldering, which reduce the thermal stress on the circuit. Another option is to incorporate thermally conductive materials, like metal-core substrates or heat spreaders, to dissipate heat more efficiently and prevent localized hot spots.
Specialized soldering equipment, such as flexible soldering irons or hot-bar soldering machines, can also be used to apply heat more precisely and minimize thermal exposure to the surrounding areas. In some cases, soldering may be performed in multiple stages, with components soldered onto local stiffeners first before being attached to the main flex circuit.
Strain Relief
Flex circuits are often subjected to repeated bending and flexing during use, which can put strain on the solder joints and components. Over time, this strain can lead to fatigue failures and compromised reliability.
To prevent these issues, strain relief features are incorporated into the flex circuit design and assembly. These features help to distribute the mechanical stress and prevent it from concentrating at specific points. Examples of strain relief features include:
- Bend relief: Gradual transitions or curved sections that reduce the stress at the point where the circuit bends
- Stiffeners: Rigid reinforcements that provide local support and stability for components or connectors
- Adhesives: Flexible adhesives that bond components to the substrate and help to distribute stress
- Mechanical fasteners: Screws, clips
Leave a Reply