Introduction to Hi-speed designs
Hi-speed designs refer to the process of creating electronic circuits, devices, and systems that can operate at very high frequencies, typically in the gigahertz (GHz) range. These designs are essential for modern applications such as high-speed communication systems, radar, and high-performance computing.
Designing hi-speed systems requires a deep understanding of the principles of electromagnetics, signal integrity, and power integrity. Engineers must consider factors such as impedance matching, transmission line effects, and power distribution to ensure that the system operates reliably at high frequencies.
Key challenges in Hi-speed designs
-
Signal integrity: At high frequencies, signals can become distorted due to reflections, crosstalk, and other effects. Designers must carefully manage these effects to ensure that signals remain clean and reliable.
-
Power integrity: High-speed circuits consume a lot of power, which can lead to voltage drops and other power-related issues. Designers must ensure that the power distribution network is properly designed to minimize these effects.
-
Electromagnetic compatibility (EMC): Hi-speed designs can generate electromagnetic interference (EMI) that can affect other nearby systems. Designers must take steps to minimize EMI and ensure that the system meets regulatory requirements.
-
Thermal management: High-speed circuits generate a lot of heat, which can affect performance and reliability. Designers must ensure that the system is properly cooled to maintain optimal performance.
Hi-speed design techniques
Impedance matching
Impedance matching is a critical technique in hi-speed designs. It involves matching the impedance of the source to the impedance of the load to minimize reflections and ensure maximum power transfer. There are several techniques for impedance matching, including:
-
Series termination: This involves adding a resistor in series with the source to match the impedance of the transmission line.
-
Parallel termination: This involves adding a resistor in parallel with the load to match the impedance of the transmission line.
-
Source termination: This involves adding a resistor in parallel with the source to match the impedance of the transmission line.
Technique | Advantages | Disadvantages |
---|---|---|
Series termination | Simple, low cost | Reduces signal swing |
Parallel termination | Maintains signal swing | Consumes more power |
Source termination | Maintains signal swing, reduces reflections | Requires additional components |
Transmission line effects
At high frequencies, the behavior of interconnects can be modeled as transmission lines rather than simple wires. This means that designers must consider factors such as characteristic impedance, propagation delay, and reflection coefficients when designing hi-speed circuits.
Some common techniques for managing transmission line effects include:
-
Microstrip lines: These are transmission lines that are fabricated on a printed circuit board (PCB) with a ground plane on one side and a signal trace on the other.
-
Striplines: These are transmission lines that are embedded within a PCB, with ground planes on both sides.
-
Coplanar waveguides: These are transmission lines that have a signal trace and ground planes on the same side of the PCB.
Technique | Advantages | Disadvantages |
---|---|---|
Microstrip lines | Simple, low cost | Susceptible to EMI |
Striplines | Good isolation, low EMI | More complex, higher cost |
Coplanar waveguides | Low dispersion, good isolation | More complex, higher cost |
Power distribution
Proper power distribution is critical in hi-speed designs to ensure that all components receive a clean and stable power supply. Some common techniques for power distribution include:
-
Power planes: These are large areas of copper on a PCB that are used to distribute power to components.
-
Decoupling capacitors: These are capacitors that are placed close to components to provide a local source of charge and minimize voltage fluctuations.
-
Voltage regulators: These are devices that provide a stable voltage supply to components, even in the presence of noise and other disturbances.
Technique | Advantages | Disadvantages |
---|---|---|
Power planes | Low impedance, good isolation | Higher cost, more complex |
Decoupling capacitors | Simple, low cost | Limited effectiveness at high frequencies |
Voltage regulators | Provides stable voltage | Higher cost, more complex |
Electromagnetic compatibility (EMC)
EMC is a critical consideration in hi-speed designs, as high-frequency signals can generate electromagnetic interference (EMI) that can affect other nearby systems. Some common techniques for managing EMC include:
-
Shielding: This involves enclosing the circuit in a conductive enclosure to minimize EMI.
-
Grounding: Proper grounding techniques can help to minimize EMI by providing a low-impedance path for high-frequency currents.
-
Filtering: This involves using filters to remove high-frequency noise from signals and power supplies.
Technique | Advantages | Disadvantages |
---|---|---|
Shielding | Effective at reducing EMI | Higher cost, more complex |
Grounding | Simple, low cost | Limited effectiveness at high frequencies |
Filtering | Effective at reducing noise | Higher cost, more complex |
Thermal management
Thermal management is a critical consideration in hi-speed designs, as high-frequency circuits generate a lot of heat that can affect performance and reliability. Some common techniques for thermal management include:
-
Heat sinks: These are devices that are attached to components to dissipate heat.
-
Fans: These are used to move air across components to remove heat.
-
Thermal vias: These are holes in a PCB that are used to conduct heat away from components.
Technique | Advantages | Disadvantages |
---|---|---|
Heat sinks | Effective at dissipating heat | Higher cost, more complex |
Fans | Simple, low cost | Limited effectiveness at high temperatures |
Thermal vias | Effective at conducting heat | Higher cost, more complex |
Examples of Hi-speed designs
High-speed communication systems
High-speed communication systems, such as 5G wireless networks and fiber-optic links, require hi-speed designs to achieve the necessary data rates and signal integrity. Some key considerations in these systems include:
-
Modulation schemes: Advanced modulation schemes, such as orthogonal frequency-division multiplexing (OFDM) and quadrature amplitude modulation (QAM), are used to achieve high data rates.
-
Equalization: Equalization techniques, such as decision feedback equalization (DFE) and feed-forward equalization (FFE), are used to compensate for channel impairments and improve signal integrity.
-
Error correction: Forward error correction (FEC) techniques, such as Reed-Solomon and turbo codes, are used to detect and correct errors in the received data.
Radar systems
Radar systems use hi-speed designs to generate and process high-frequency signals for object detection and tracking. Some key considerations in these systems include:
-
Pulse compression: Pulse compression techniques, such as chirp and phase coding, are used to improve range resolution and signal-to-noise ratio.
-
Beamforming: Beamforming techniques, such as phased arrays and digital beamforming, are used to steer the radar beam and improve angular resolution.
-
Doppler processing: Doppler processing techniques are used to measure the velocity of moving targets.
High-performance computing
High-performance computing systems, such as supercomputers and data centers, require hi-speed designs to achieve the necessary processing speeds and data throughput. Some key considerations in these systems include:
-
Parallel processing: Parallel processing techniques, such as multi-core processors and graphics processing units (GPUs), are used to achieve high processing speeds.
-
Interconnects: High-speed interconnects, such as PCI Express and InfiniBand, are used to transfer data between processors and memory.
-
Cooling: Advanced cooling techniques, such as liquid cooling and immersion cooling, are used to remove heat from high-performance computing systems.
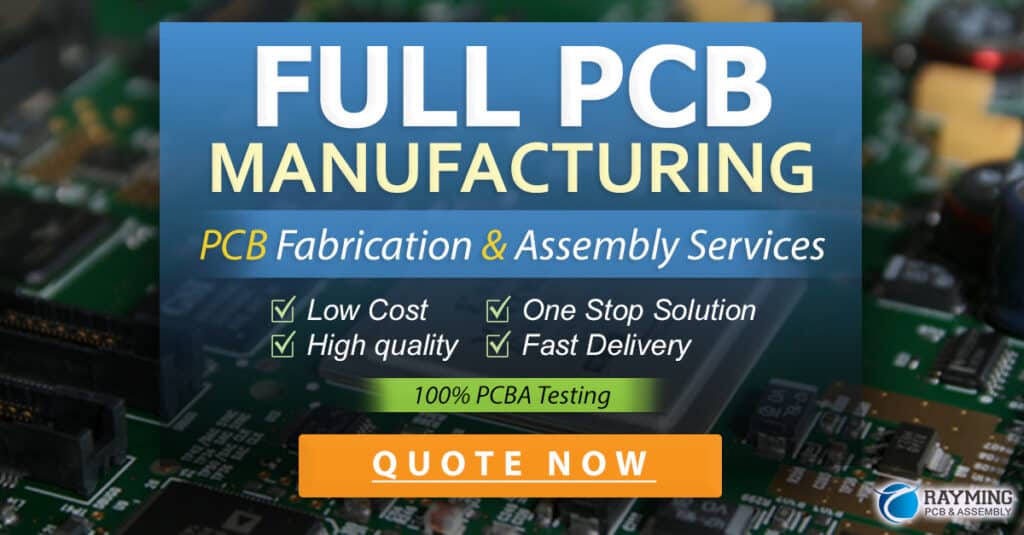
Frequently Asked Questions (FAQ)
What is the difference between hi-speed designs and regular designs?
Hi-speed designs are specifically designed to operate at very high frequencies, typically in the gigahertz (GHz) range. Regular designs, on the other hand, are designed for lower frequencies and may not be suitable for high-speed applications.
What are some common challenges in hi-speed designs?
Some common challenges in hi-speed designs include signal integrity, power integrity, electromagnetic compatibility (EMC), and thermal management. These challenges arise due to the high frequencies and power levels involved in hi-speed designs.
What is impedance matching and why is it important in hi-speed designs?
Impedance matching is the process of matching the impedance of the source to the impedance of the load to minimize reflections and ensure maximum power transfer. It is important in hi-speed designs because reflections can cause signal distortion and degrade performance.
What are some common techniques for managing transmission line effects in hi-speed designs?
Some common techniques for managing transmission line effects in hi-speed designs include using microstrip lines, striplines, and coplanar waveguides. These techniques help to control the characteristic impedance and propagation delay of the transmission lines.
What is electromagnetic compatibility (EMC) and why is it important in hi-speed designs?
Electromagnetic compatibility (EMC) refers to the ability of a system to operate without causing or being affected by electromagnetic interference (EMI). It is important in hi-speed designs because high-frequency signals can generate EMI that can affect other nearby systems.
Conclusion
Hi-speed designs are essential for modern applications such as high-speed communication systems, radar, and high-performance computing. Designing these systems requires a deep understanding of the principles of electromagnetics, signal integrity, and power integrity.
Engineers must consider factors such as impedance matching, transmission line effects, power distribution, EMC, and thermal management to ensure that the system operates reliably at high frequencies. By using advanced techniques such as microstrip lines, decoupling capacitors, shielding, and parallel processing, designers can create hi-speed systems that meet the demanding requirements of today’s applications.
As technology continues to advance, the demand for hi-speed designs will only increase. Engineers who are skilled in hi-speed design techniques will be well-positioned to meet this demand and create the next generation of high-performance electronic systems.
Leave a Reply